The current standard of care for oral rehabilitation using implants focuses not only on restoring the function of missing teeth but also on achieving satisfactory esthetics (Pimkhaokham et al. 2024). To meet these goals, precise implant placement based on prosthetically driven decision-making is essential. Since its inception in the mid-1990s, computer-guided implant surgery has rapidly gained traction (Fortin et al. 2006). The introduction of cone beam computed tomography (CBCT) has further enhanced this process by providing detailed 3D imaging of the jawbone at a reasonable cost and with minimal radiation exposure (Tahmaseb et al. 2018). This technology enables the preoperative collection of extensive data, such as bone volume and quality, the location of important anatomical structures, and the identification of any pathologies, all of which are crucial for successful rehabilitation (D’haese et al. 2012). Computer-aided methods provide significant benefits in implant treatment planning, enabling clinicians to perform successful implant-based rehabilitations while minimizing or even eliminating the need for large mucoperiosteal flap elevations (Carosi et al. 2021). This results in less pain and discomfort for patients. When operating freehand, surgeons often raise large mucoperiosteal flaps to improve visibility of the implant site. However, this may be reduced, as the surgeon can pre-visualize the recipient site and use computer-guided technology to place the implant accurately (Block & Emery 2016). Freehand implant placement is always challenging for clinicians due to clinical factors such as patient movement during drilling, limited visibility of the surgical site, especially in the posterior zone, and the need to interpret and translate two-dimensional radiographs into a three-dimensional surgical field. (Pozzi et al. 2014). The surgeon is required to make multiple decisions – from surgical to prosthetic – to achieve optimal 3D implant positioning within a short time frame to integrate the esthetic, biomechanical, and functional aspects. Comprehensive preoperative planning can ease this pressure, giving the surgeon more opportunity to focus on the patient and manage tissue handling with greater care.
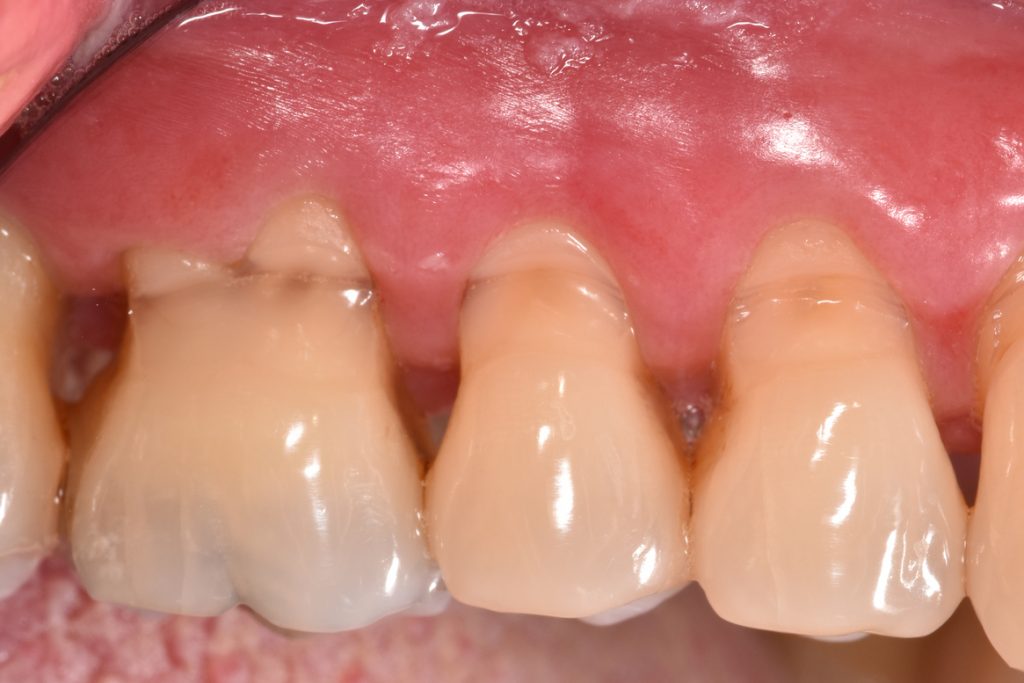
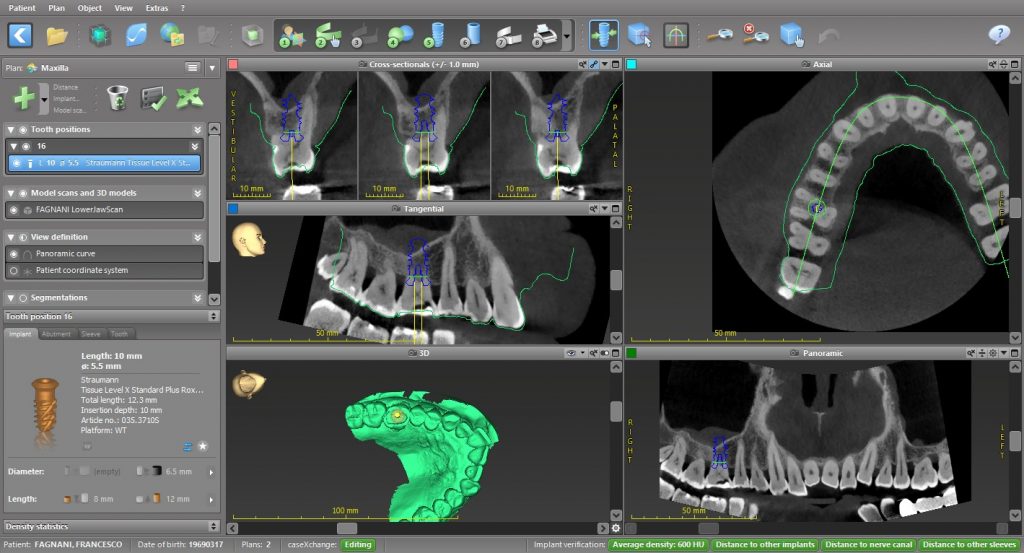
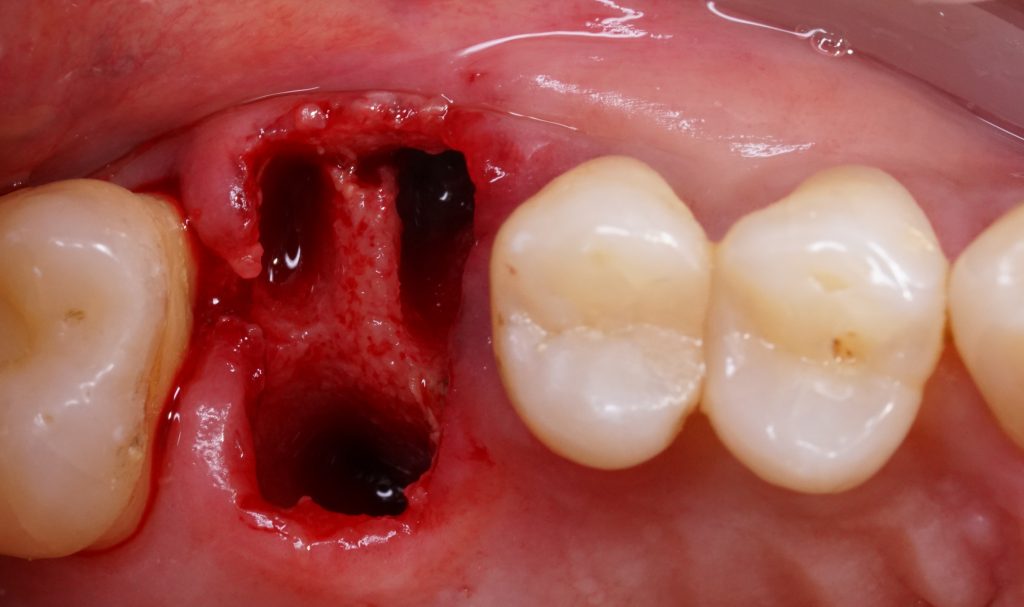
Static guided surgery
The core concept of static computer-assisted implant surgery (CAIS) is straightforward: an acrylic “template” is used to guide drills precisely to the desired osteotomy site for implant placement. This method relies on a 3D-printed surgical guide featuring “sleeves” that control the angle and position of the drills, along with “stops” to regulate depth (Carosi et al. 2022). The guide can be supported by teeth, oral mucosa, underlying bone through mini screws, or a combination of these. It can be a single-piece guide or “stackable” for multiple procedures, such as ostectomy before implant placement or lateral sinus floor augmentation.
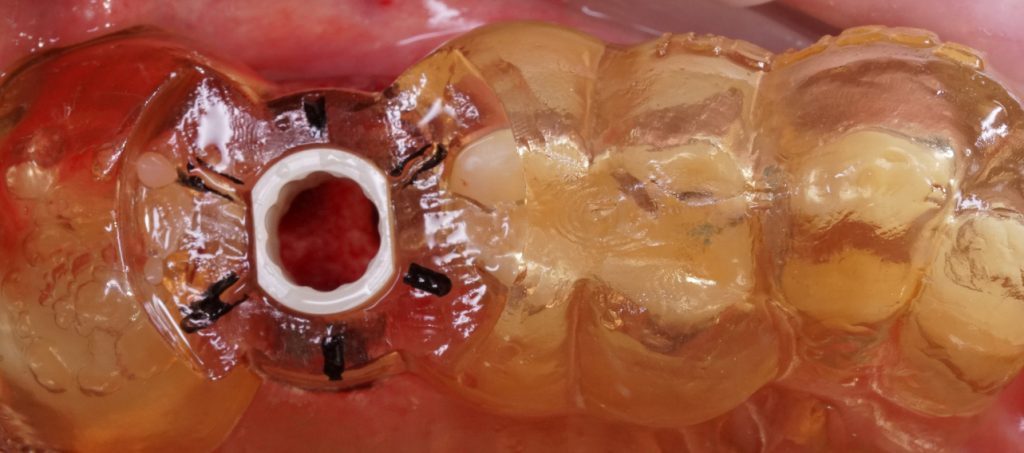
However, the principle of static CAIS is where its simplicity ends, and practical application begins. Today, nearly every major implant brand offers its own guided surgery software, instruments, and protocols. This diversity stems from each company’s unique philosophy, design, historical features, and perspective on implant surgery, rather than evidence-based practice.
Computer-assisted implant positioning involves both static and dynamic systems. Static guided surgery refers to fixed implant placement determined in advance, without live visualization during the preparation of the implant site. Studies have shown that tooth-supported templates provide more accurate implant placement compared to mucosa- or bone-supported ones. However, for fully edentulous patients, mucosa- or bone-supported templates are used and secured to the jaws with anchor pins. Bone-supported templates require a full-thickness mucoperiosteal flap to stabilize them, while mucosa-supported templates can be fixed without raising a flap, allowing for flapless implant placement. This reduces patient discomfort, surgery time, and healing duration. Nevertheless, mucosal resilience and muscle tension can affect the fit of mucosa-supported templates, potentially reducing placement accuracy during flapless procedures (D’haese et al. 2017). Despite this, the flapless approach for completely edentulous arches using mucosa-supported templates may reduce overall treatment complexity, costs, time, and patient discomfort. However, accuracy is not the only critical factor. The ease of use, reliability of the entire workflow, availability of various drills and components, durability, and cost also play an important role. Instead, numerous protocols exist, each with distinct results, potential benefits, and limitations (Wismeijer et al. 2018).
Dynamic
Dynamic CAIS systems operate on principles similar to GPS navigation. In earlier generations, these systems used stereoscopic cameras to continuously track the position of fiducial markers on both the patient and the surgeon’s handpiece. The software would then calculate the precise location of the patient and the handpiece in space and project the drills onto the patient’s CBCT images in real time via a screen. This setup required maintaining accurate spatial alignment between the patient and the drills, necessitating custom-made splints for both CBCT and surgery, as well as detailed registration and calibration before the procedure (Pozzi et al. 2021). Dynamic guided surgery, or navigation, enables real-time visualization during implant site preparation while the drills are in use. Unlike static systems, it does not require a template, but a marker placed in the oral cavity fixed to the teeth or to the bone far from the surgical site, allowing for an unobstructed view of the surgical area and easier management of soft tissues. With dynamically assisted full surgical guidance, deviations from the preplanned implant position can be detected and corrected during the procedure. The original concept of dynamic navigation has evolved to integrate both surgical and prosthetic aspects, aiming to achieve precise, site-specific outcomes and meet patient expectations for a natural appearance, including the provision of an immediate temporary fixed dental prosthesis (FDP) (Pozzi et al. 2024).
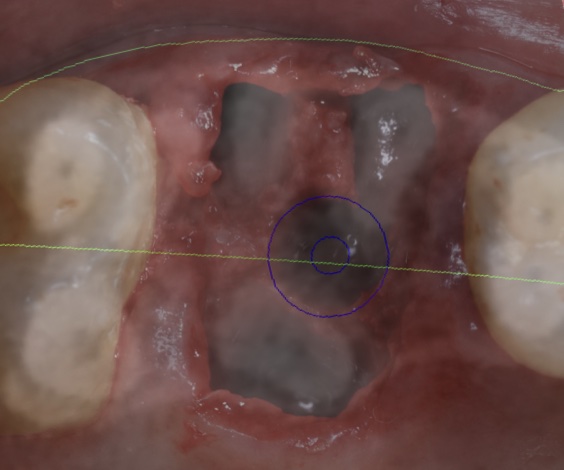
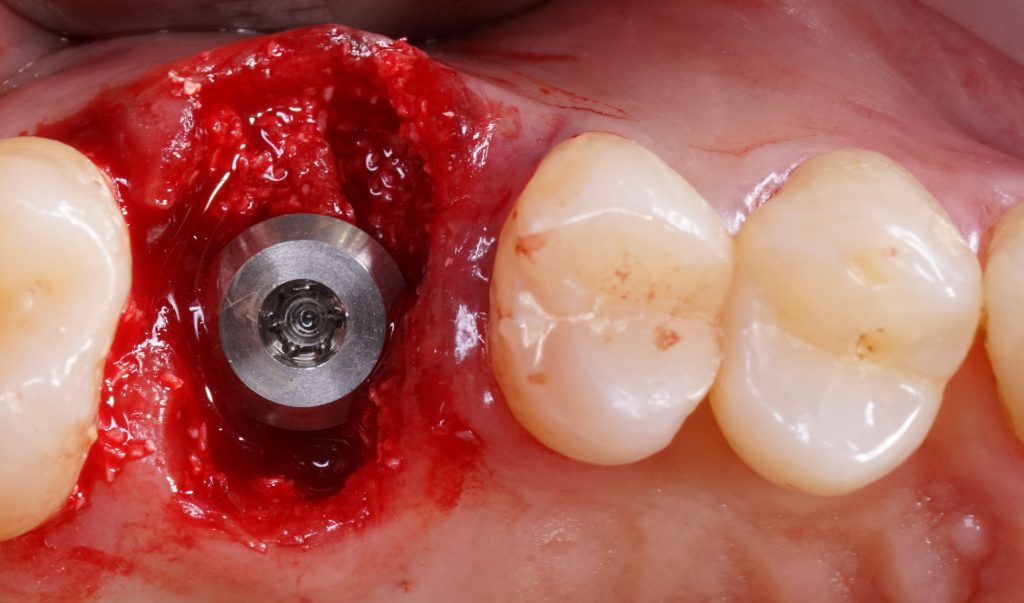
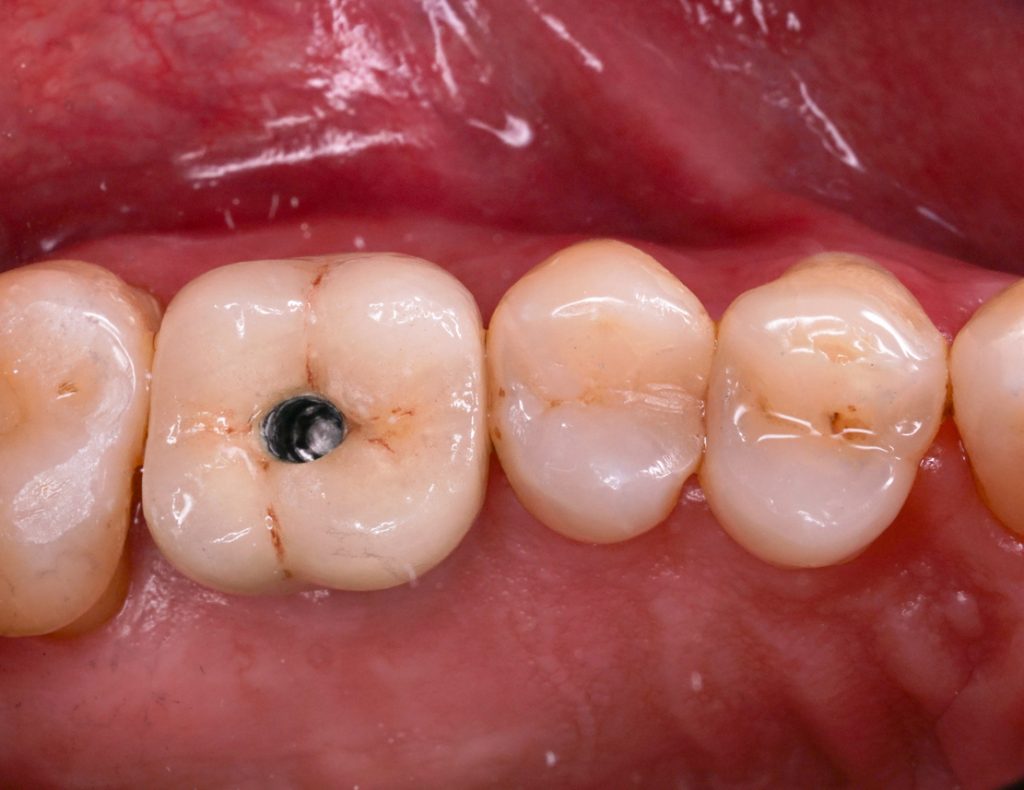
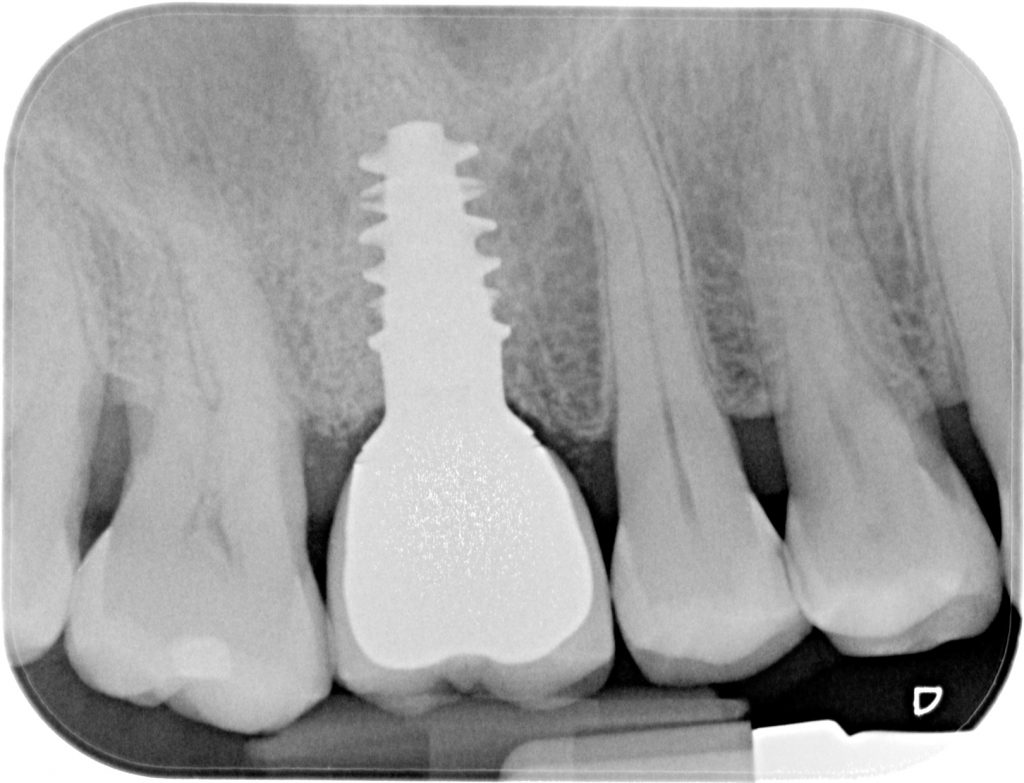
Robotic
Robotics in implant surgery was gradually introduced to try to reduce the deviations from pre-planned to actually positioned implants using s-CAIS and d-CAIS techniques to perform clinical procedures (Li et al. 2023). The r-CAIS integrated many advanced technologies, such as artificial intelligence (AI), machine vision, multi-sensor information dataset fusion and 3D graphics visualization (Zhang et al. 2024). Yang et al. (Yang et al. 2017) classified six levels of autonomy for medical robotics. The r-CAIS involved the use of an operator-controlled (level 1 or semi-active) or an autonomous robotic arm (level 2 or ADIR) for both osteotomy preparation and implant placement (Wang et al. 2024). In level 1, the robot offered semi-active control, providing mechanical support or guidance to the surgeon who is in control of the robot’s actions. The Level 1 r-CAIS setup entailed the robot managing angular adjustments while the surgeon oversaw the speed and force applied during drilling and implant insertion. The robotic arm was driven by the operator in and out of the patient’s mouth to and from the implant recipient site. In level 2, the robot had autonomy in drilling and implant insertion. It can execute specific predefined subtasks while the surgeon assists and provides a backup control through a dedicated computer screen (Shi et al. 2024). The robotic arm enters the patient’s mouth up to the target coordinates and exits following the reverse trajectory in an autonomous manner. The autonomous movements of the robot rely on various factors: (i) the surgeon establishes the initial and final points for the robot’s motion based on information from the bone and mucosa within the software; (ii) the robot and jaws are connected within the infrared visual space; (iii) the robot executes surgical procedures following a predetermined path under the guidance of infrared vision (Li et al. 2023). Autonomous dental implant robotic surgery minimizing the operator influence may execute accurate implant drilling and placement under visual-force feedback cooperative control, standardizing clinical performance regardless of the type of edentulism, type of jaw, or site characteristics. In level 2, an autonomous robotic arm enters the patient’s mouth advancing up to the target coordinates and exiting along the reverse trajectory in an autonomous manner. ADIR surgery excludes the operator influence on the accuracy outcome, maintaining the full autonomy in trajectory control, drilling speed and implant insertion, while the surgeon assists and provides backup control through a dedicated computer screen.
Discussion
Over the past few years, there has been a growing trend in medical robot technology towards executing tasks of greater complexity with an increased level of autonomy. R-CAIS technology can be considered similar to d-CAIS since there is no physical template guiding the clinician or the robot in the surgical phases. A recent systematic review of d-CAIS by Yu et al. (Yu et al. 2023) reported encouraging data of mean global platform deviation, global apex deviation, and angular deviation (1.07 mm (95% CI: 0.96 to 1.17), 1.27 mm (95% CI: 1.06 to 1.47), and 3.43° (95% CI: 2.94 to 3.93). Moreover, a recently published prospective clinical trial (Pozzi et al, 2024) evaluating the accuracy of d-CAIS for complete-arch restorations reported overall platform, apex and angular deviations of 1.17 mm (SD 0.57 mm), 1.30 mm (SD 0.62 mm) and 2.19° (SD 1.26°), respectively. The early findings of the literature showed that ADIR performed better in terms of accuracy, dramatically reducing the 3D linear and angular deviations. This may be related to the capability of ADIR to automatically adjust for slight movements of the patient’s head by continuously capturing real-time positional data through optical tracking of the patient tracking array. In the same way, any deviations experienced during implant site preparation were immediately addressed through autonomous adjustment of the drilling and, where needed, a reset and re-calculation of the drilling trajectory.
A recent RCT by Yotpibulwong et al. investigating the accuracy of dynamic and static guided implant placement in single tooth gaps reported deviations at platform (mm) of 1.06 ± 0.67 and 1.02 ± 0.45, respectively, at apex (mm) 1.40 ± 0.71 and 1.28 ± 0.50, respectively, and angular deviation (degrees) of 3.18 ± 2.04, 3.28 ± 1.57, respectively. A recent RCT by Yimarj et al. reported accuracy of static and dynamic CAIS in partially edentulous patients, deviations at implant platform of 1.04 ± 0.67 and 1.24 ± 0.39 mm, at apex 1.54 ± 0.79 and 1.58 ± 0.56 mm, and angular 4.08° ± 1.69° and 3.78° ± 1.84°, respectively. Two RCTs by Yimarj and Yotpibulwong showed that dynamic or static guided surgery may optimize implant placement, experiencing linear deviations at platform and apex of less than 2 mm without significant differences between the two technologies .(Yotpibulwong et al. 2023) .(Yimarj et al. 2020). However, the data reported in the literature showed consistently higher accuracy of ADIR implant placement despite different clinical scenarios. From single tooth gap to complete arch restoration, implants placed by means of ADIR reported lower mean global and angular deviations from the pre-planned 3D coordinates to the actual implant positioning compared to the s-CAIS and d-CAIS. Moreover, all the clinical studies investigating ADIR reported low values of SD, demonstrating that ADIR could be feasible for implant placement with accuracy unrelated to the type of edentulism or type of arch. Such positive outcomes resulted from ADIR addressing the intrinsic limitations of the different CAIS techniques. S-CAIS required a wide mouth opening due to the need to use a dedicated drill kit with “sleeve extension” length, 3D printed template production and seating in the patient mouth might contribute to increase overall surgical deviations. The inability of s-CAIS to detect and correct the drilling path for any type of deviation may contribute to experience such inaccuracy in respect to r-CAIS.
In regard to complete arch s-CAIS accuracy, a recent systematic review showed an average global platform deviation, global apex deviation, and angular deviation of 1.23 mm (95% CI 0.97-1.49), 1.46 mm (and 95% CI 1.17-1.74) and 3.42° (95% CI 2.82-4.03) (Carosi et al. 2022).
In contrast, r-CAIS further improved on the clinical performance of d-CAIS, dynamically tracking the motion of the dynamic reference frame (DRF) firmly secured to the patient’s anatomy, and computing tracked data in real time, simultaneously adjusting the drilling trajectory in an autonomous manner. ADIR did not need operator handling of the surgical handpiece as does d-CAIS, because the robotic arm firmly managed the drilling path. Moreover, autonomous robotic implant surgery did not require any information displayed in real time on a screen as do the navigation systems. Such d-CAIS real time alignment between visual and tactile feedback and the related brain response, potentially lead to deviations from the planned coordinates and oblige the operator to focus attention on the screen instead of the surgical field. Moreover, the navigation-assisted surgical drilling required a learning curve and its accuracy could be influenced by surgeon training and expertise.
Conclusions
Currently, there is no definitive evidence showing a difference in accuracy outcomes between static and dynamic CAIS, although recent meta-analyses have indicated that dynamic CAIS (d-CAIS) may lead to a greater reduction in angular deviation. Future studies with larger sample sizes and different outcome measures, other than average deviation, may help to clarify these potential differences. For now, the decision to use static or dynamic CAIS should be based on local anatomical, patient, and operational factors rather than expected accuracy differences. Combining static and dynamic CAIS could enhance implant placement accuracy for both single gaps and edentulous patients, but this approach involves substantial operational and cost implications, raising questions about its cost effectiveness. There is a strong need for research to explore the link between increased accuracy levels and clinical as well as patient-reported outcomes, evaluate actual patient benefits, and assess the cost efficiency of these technologies. Robotic CAIS is likely to gain more attention soon, as case series have shown that it offers greater placement accuracy compared to human-operated CAIS, even in complex scenarios like edentulous arches. However, the high costs, complexity, and the need for human intervention and direct supervision limit its broader adoption. In the future, expanding robotic arms to other surgical and non-surgical procedures could enhance their applicability and benefits. Additionally, advancements in machine learning and artificial intelligence may pave the way for more autonomous robotic surgical applications, potentially increasing their use in implant dentistry.