Introduction
Following tooth loss, the alveolar ridge will undergo dimensional changes (Araujo et al. 2005; Cardaropoli et al. 2003) that can lead to horizontal and vertical bone defects. This can lead to complex clinical situations regarding implant placement and treatment planning, mainly when dealing with anterior esthetic areas requiring bone augmentation procedures (Benic & Hammerle, 2014).
Different bone regenerative techniques have been proposed and tested for reconstructive surgery of deficient alveolar ridges to allow adequate bone for dental implant placement. Current bone augmentation procedures have shown high effectiveness, reconstructing bone with a mean horizontal bone gain of 4.3 mm (Sanz-Sanchez et al. 2015) and a range between 3.5 and 4.2 mm in a vertical aspect (Urban et al. 2019). However, bone grafting procedures still have a high complication rate, ranging from 0-45%, with a mean of 16.8%, and the need to regraft from 0% to 23% of treated sites (Sanz-Sanchez et al. 2022).
These bone augmentation techniques, although predictable, are highly technique-sensitive procedures with high post-surgical morbidity that can have direct implications for the patient’s quality of life, mostly when complications arise that can jeopardize the success of the augmentation procedure. Thus, it has been recommended that these procedures be improved, techniques or materials be further developed in attempts to lower complication rates and performed with less invasive surgical interventions (Sanz-Sanchez et al. 2022). Hence, it is not surprising to note that the 15th European Symposium in Bone Regeneration recommended future research in the additive manufacturing of biomaterials to create patient-friendly customized scaffolds to fit the bone defect anatomy perfectly (Sanz et al. 2019)
During the last decade, new technologies have been intensely implemented throughout the different fields of dentistry. In the field of implantology, incorporating these technologies to place implants predictably through guided surgery has been widely supported by the literature (Wismeijer et al. 2018). However, limited evidence or scientific support is available when evaluating the added value of implementing these technologies into implant cases requiring bone augmentation, with only a recently published narrative review about this topic (Tommasato et al. 2024). In other fields of medicine, like maxillofacial or reconstructive surgery, 3D technologies are commonly used to facilitate bone augmentation procedures and their predictability (Mehra et al. 2011).
This review aims to provide a comprehensive overview of the various opportunities that the implementation of 3D digital technologies and workflows offers to the field of bone augmentation in implant dentistry. It discusses how these advancements can enhance the predictability of bone augmentation procedures, improve treatment outcomes, shorten surgical duration, improve the patient experience, and minimize postoperative morbidity. Specifically, the review describes the following topics:
- Simultaneous application of 3D guided implant placement surgery with bone augmentation techniques.
- Utilization of 3D stereolithographic models to evaluate defect morphology, pre-customize bone augmentation membranes such as PTFE membranes or ti-meshes, and digitally plan the volume of reconstruction.
- Digital guidance for autologous bone block harvesting and for the preparation of lateral sinus lift windows.
- The use of 3D-customized scaffolds for guided bone augmentation (GBR) procedures (titanium, PEEK, other materials)
Guided implant surgery and horizontal bone augmentation
According to the Benic & Hammerle classification, defects that require horizontal bone augmentation can be classified from 0 to 5, and based on this, the indicated treatment option is either implant placement with simultaneous horizontal bone augmentation or a staged approach. The recommended treatment is a simultaneous approach when treating class 0-3 bone defects and a staged approach for class 4-5 defects (Benic & Hammerle, 2014). However, the predictability of bone regeneration also depends on other factors that need to be evaluated, such as the anatomy of the bone defect (Yu et al. 2023) and the amount of implant expected to be exposed and the need for horizontal GBR and vertical thread coverage.
When systematically evaluating the effectiveness of simultaneous lateral bone augmentation and implant placement, it was determined that these interventions significantly reduced the defect height in the simultaneous approach. The most frequently reported and supported treatment option was simultaneous GBR with a xenograft and an absorbable membrane, which had a weighted mean difference (WMD) of 4.42 mm of buccal dehiscence reduction (Sanz-Sanchez et al. 2015) with a complication rate ranging from 16-22% and a mean implant survival rate of 95% after a mean follow-up of 76.5 months (Thoma et al. 2019).
However, a critical and often overlooked factor in the literature is the presence of horizontal bone deficiencies at the implant site, which can hinder the clinician’s ability to achieve proper free-hand implant placement in the ideal, prosthetically driven position. In such scenarios, guided implant placement proves to be invaluable, offering enhanced accuracy in both the linear and angular positioning of the implant, as evidenced by Romandini et al. (2023). This method is particularly beneficial in challenging cases, such as the placement of immediate implants where precision is paramount, as highlighted by Chen et al. (2018). Additionally, meticulous digital planning is instrumental towards assessing the extent of implant exposure post-placement, allowing the clinician to gauge the feasibility of concurrent bone augmentation based on the residual defect height (Sanz-Sanchez et al. 2015) as well as the defect characteristics and surrounding anatomy (Yu et al. 2023) (Figures 1, 2, and 3).
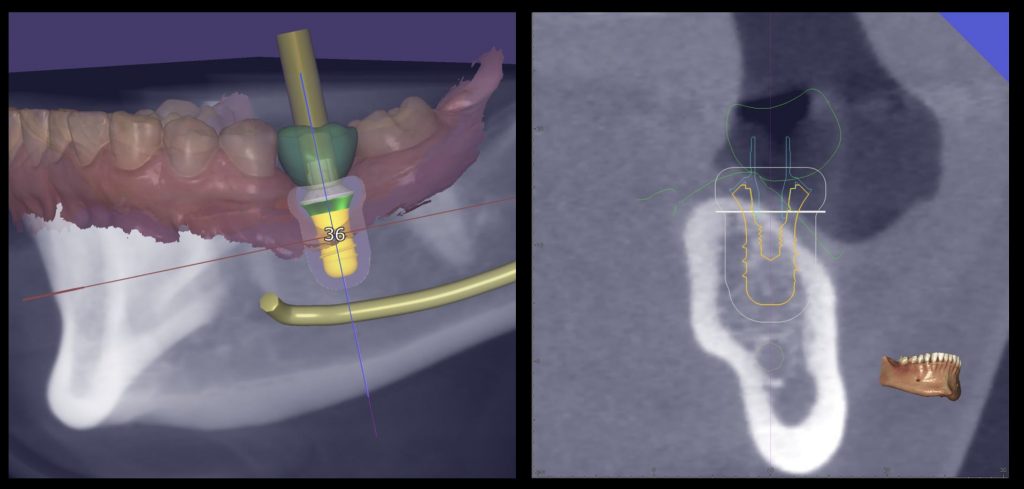
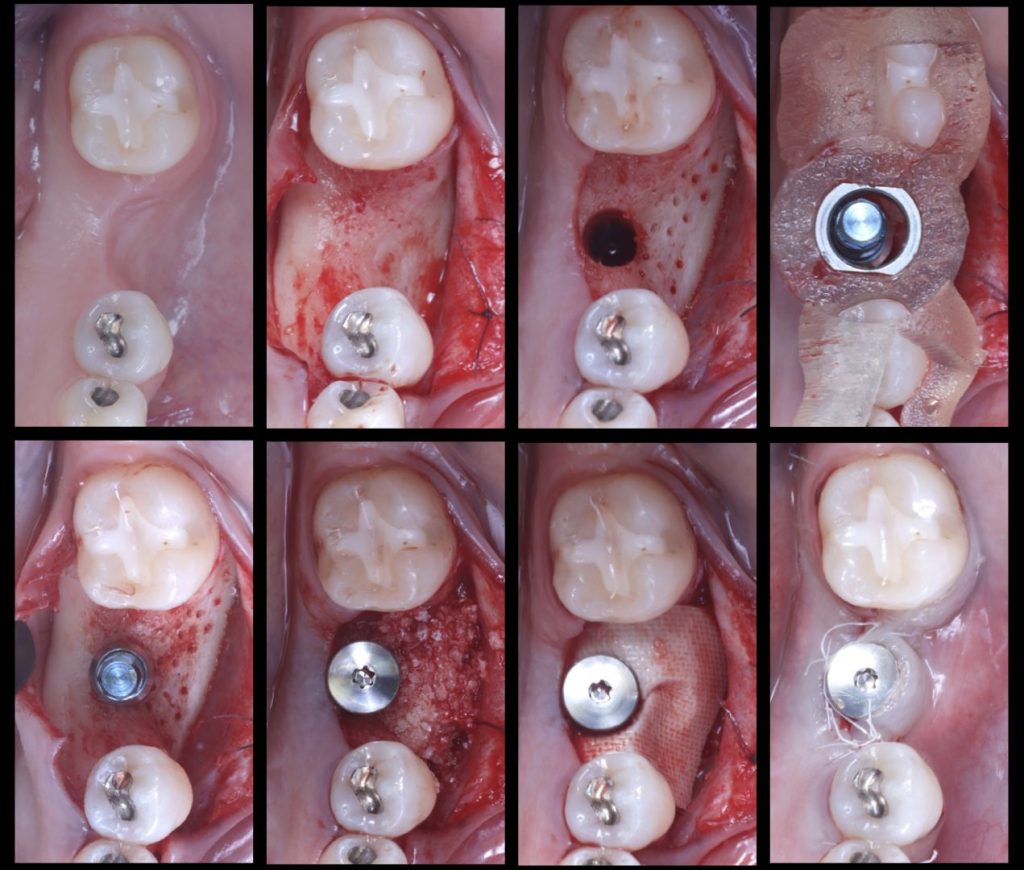
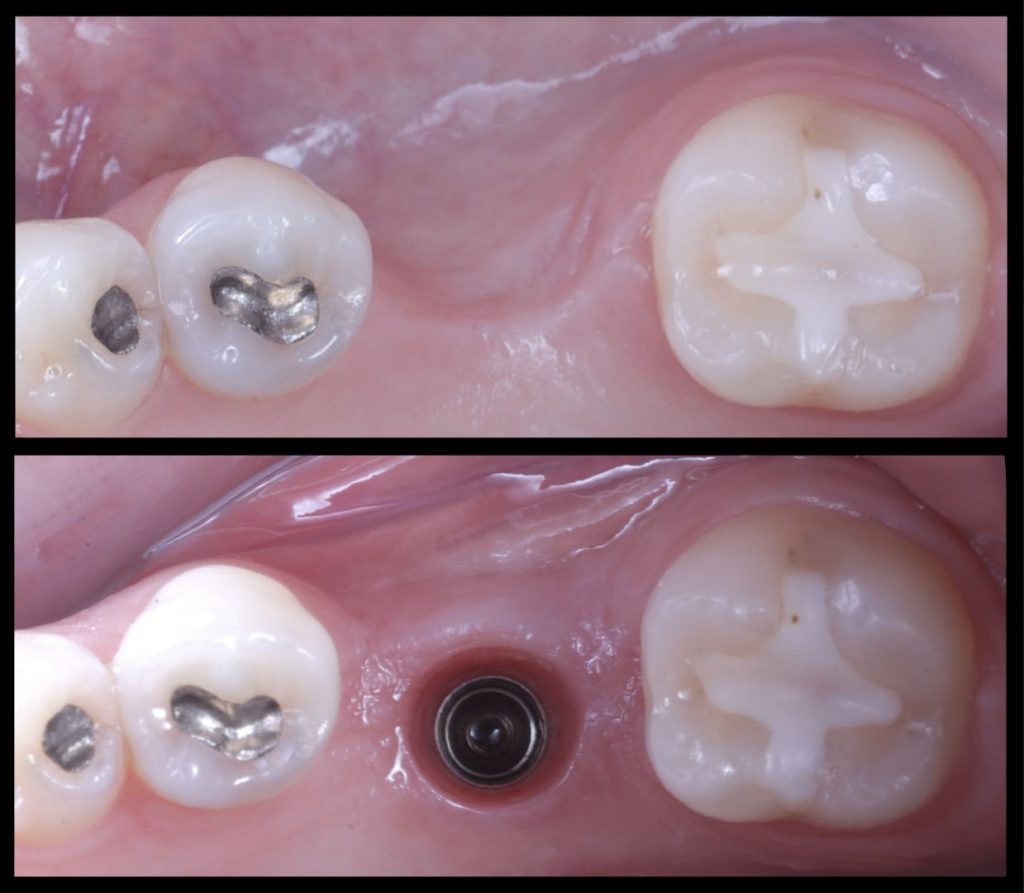
Use of 3D stereolithographic models
In cases where staged bone augmentation and implant placement are needed, guided bone regeneration with either absorbable or non-resorbable membranes is a widely documented procedure for bone augmentation (Urban & Monje, 2019). With this treatment option, the most challenging step of the surgery remains the part of trimming, adapting and fixing the membrane to adjust the required volume of necessary bone augmentation and its posterior fixation.
The implementation of 3D printers has had an impact on current dentistry protocols and allows the printing of precise 3D models very simply and quickly. Within maxillofacial surgery, 3D stereolithographic models for reconstructive surgery have been widely used, improving the predictability of clinical outcomes compared to similar treatments without its use (Mehra et al. 2011). Some advantages this can offer are the direct visualization of anatomical structure, creation of surgical guides and templates, adaptation or pre-bending of plates/meshes/membranes, reduction of surgical time, and open wound exposure, all leading to more uneventful healing and more predictable results (Figure 4).
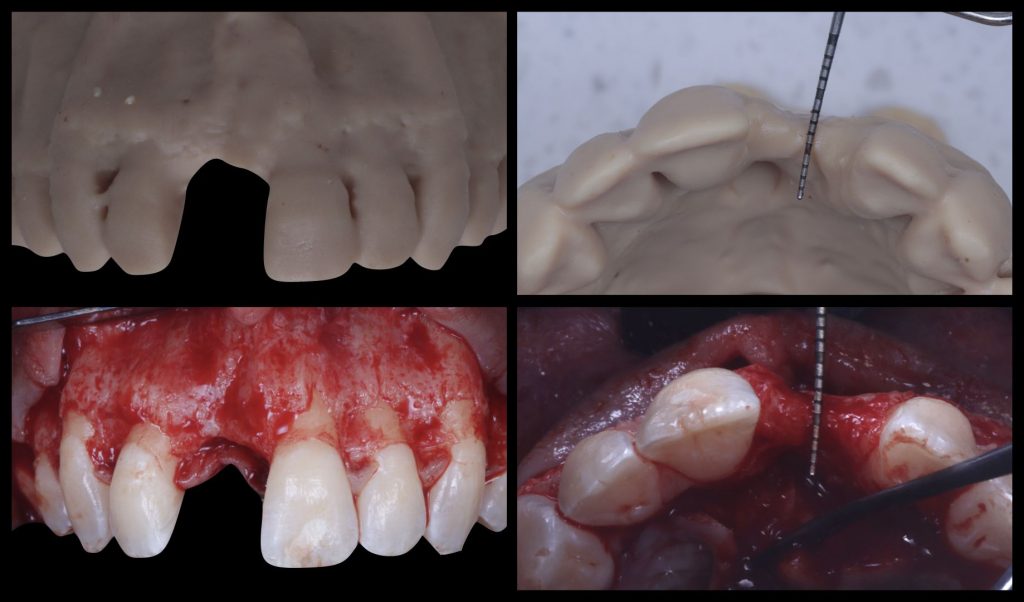
Stereolithographic models can be used in different ways when performing bone augmentation. The most straightforward option is to obtain a 3D segmentation of the patient’s maxilla/mandible anatomy including the bone defect, and after printing it, adapting, trimming or pre-bending the desired membrane (native collagen, PTFE, ti-mesh) to the required anatomy of the defect. This reduces the manipulation of the membrane and the possibility of contamination, which is especially relevant for non-resorbable membranes. Furthermore, this leads to an overall reduction in the surgical complexity, surgical time and subsequent improvement of the patient’s post-operative quality of life.
Another approach that has been described, based on the 3D segmented surface of the patient’s maxilla or mandible, is to perform a virtual ridge augmentation of the atrophic area. The amount of additional bone volume needed can be determined based on the ideal implant position, following the already planned final prosthetic outcome and the prosthetically guided GBR concept (Chiapasco & Casentini, 2018). Once the digital bone augmentation is planned, it can be turned into a 3D printed model and used intrasurgically to adapt the membrane of choice, reducing surgical time and potential errors (Al-Ardah et al. 2018). For this purpose, the models must be printed with resins that can be sterilized and used during surgery without contaminating the regeneration materials (Figures 5, 6).
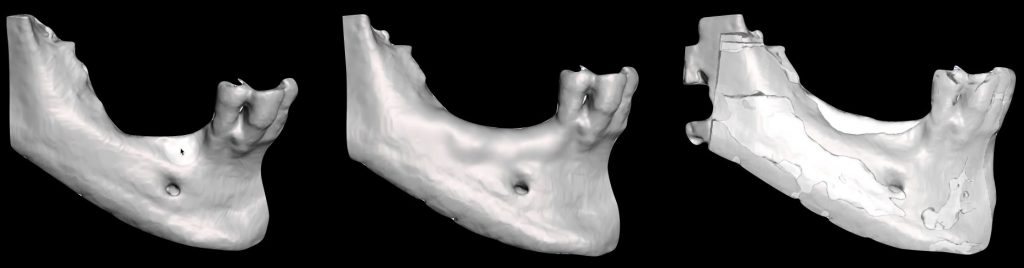
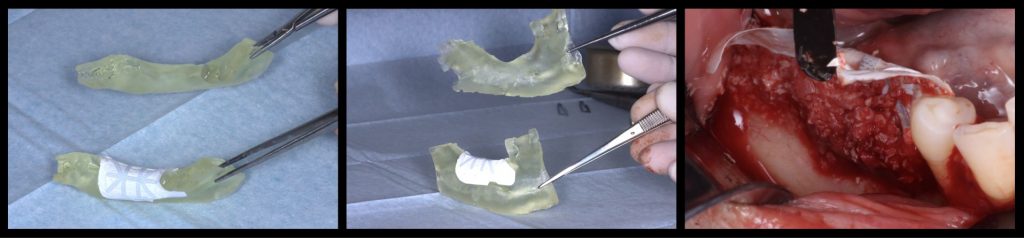
Digitally guided bone block harvesting and lateral window preparation
Bone augmentation by means of autologous intraoral bone blocks has been a widely described technique, whether they are used as onlay block grafts (Chiapasco et al. 2020), Khoury technique (Khoury & Hanser, 2015), or Wafer technique (Merli et al. 2017). The biological properties of autologous bone make it one of the most predictable materials for bone reconstruction. All these techniques have a common factor: an autologous bone block, most commonly obtained from the mandibular ramus or, in some cases, from the mandible symphysis.
However, bone block harvesting is a complex surgical procedure that demands surgical expertise due to its inherent challenges, such as limited donor bone availability, potential inadequacy of harvested bone volume for the required augmentation, risk of nerve or dental root injury, and increased postoperative patient discomfort. To overcome these limitations, advancements in digital technologies have paved the way for a computer-guided approach to bone block harvesting, enhancing its predictability. This innovative approach involves designing a custom template that can be fabricated with a 3D printer and utilized during surgery. The use of this template contributes to a quicker, safer, and more accurate bone harvesting process, ensuring the optimization of autologous bone yield (De Stavola et al. 2015) (Figures 7,8,9).
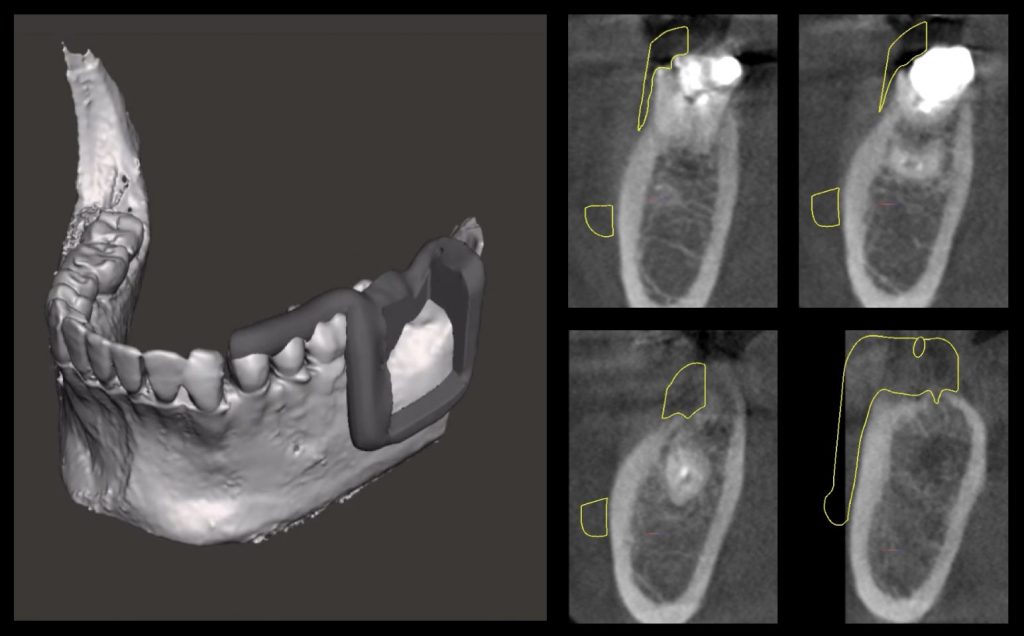
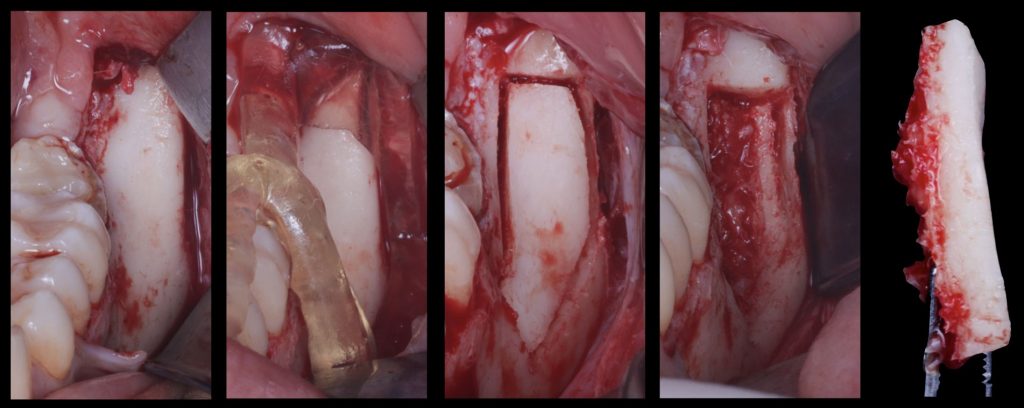
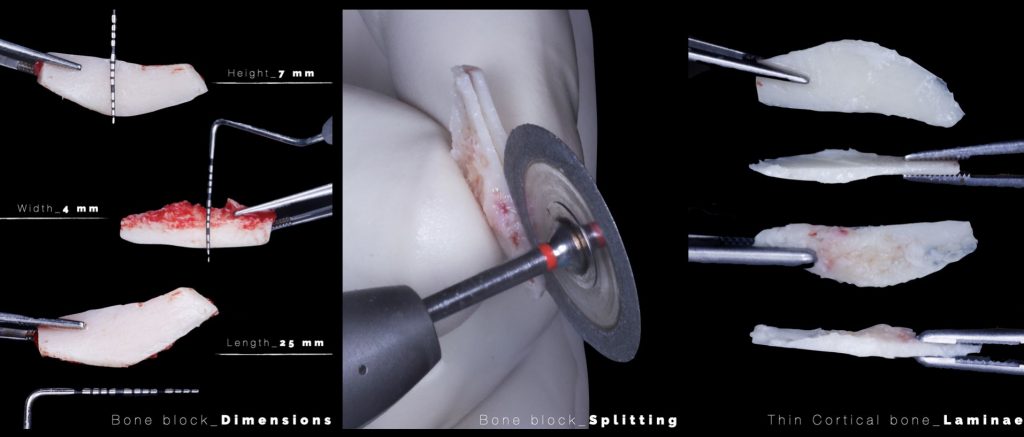
3D customized scaffolds for gbr
The use of titanium meshes as scaffolds for GBR was first described in the 90s as a novel method for localized vertical and horizontal augmentation of the alveolar ridge, due to its tissue compatibility and capacity for autogenous bone graft stabilization and integration (von Arx et al. 1996). Years later, a clinical trial comparing the use of autologous block grafts alone or in association with a titanium mesh for ridge augmentation found that onlay grafts protected by a ti-mesh showed significantly less graft resorption (Test: 0.9 mm vs. Control: 1.9 mm), making it a more effective option (Roccuzzo et al. 2007). A more recent randomized control trial comparing GBR possibilities in the posterior mandible found that a ti-mesh covered with a collagen membrane was just as effective as a d-PTFE membrane. No significant differences between groups regarding the vertical bone gain, complication rate, or implant stability were found (Cucchi et al. 2017).
However, while ti-meshes boast beneficial properties such as elasticity, the ability to conform to various defect shapes, impressive mechanical characteristics, and excellent biocompatibility, they do have a relatively high mean exposure rate of 11% (Sabri et al. 2024), which exceeds that of many similar GBR procedures. Part of these issues may arise from the manual bending and shaping required to fit the mesh precisely to the defect, a process that can be time-consuming and may increase surgery duration. Manipulating the ti-mesh also heightens the risk of infection due to prolonged contact with surgical gloves and surfaces. Moreover, if the ti-mesh is not adequately contoured and sharp edges are left exposed, they can mechanically irritate the overlying flap during healing, potentially leading to early or late exposure (Xie et al. 2020).
To overcome these limitations and leverage contemporary digital technologies, 3D customized titanium meshes have been developed. These meshes retain all the mechanical and biological advantages that standard ti-meshes provide for GBR, while simultaneously mitigating their drawbacks. Being tailored to the precise anatomy of the patient’s defect, these custom meshes can significantly decrease surgical time, reduce patient morbidity, and minimize the risk of post-operative complications (Figures 10, 11, 12).
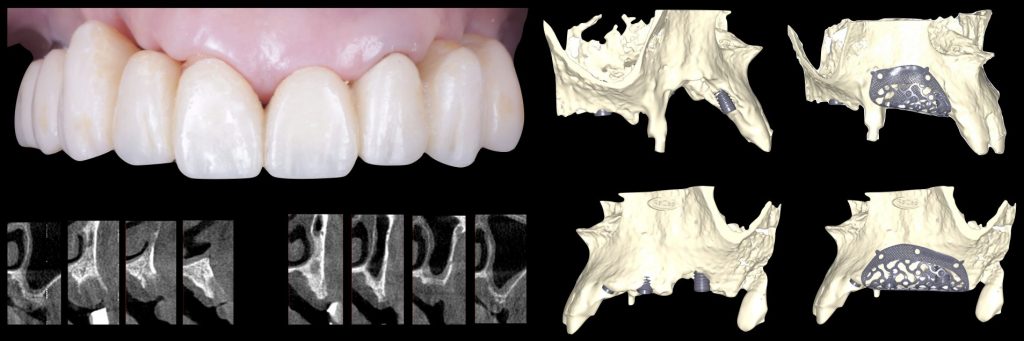
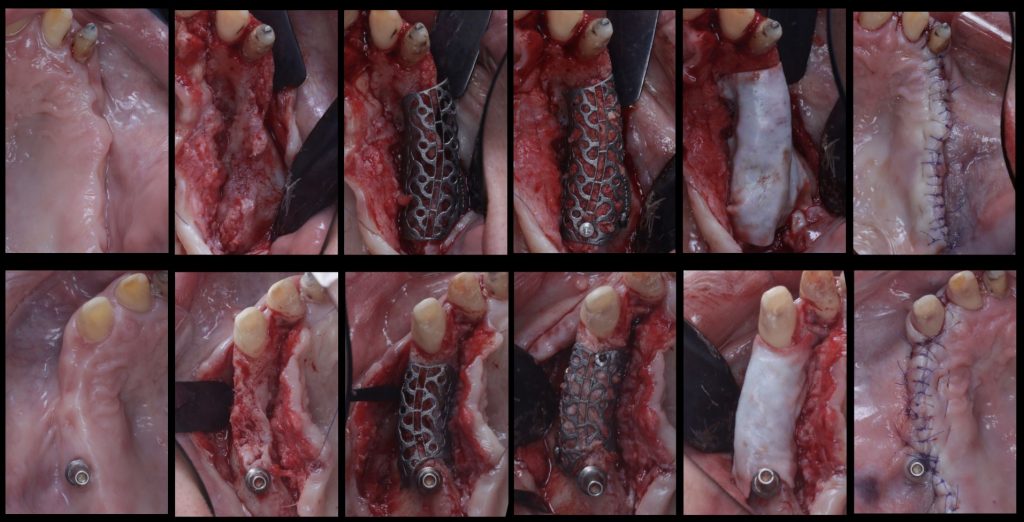
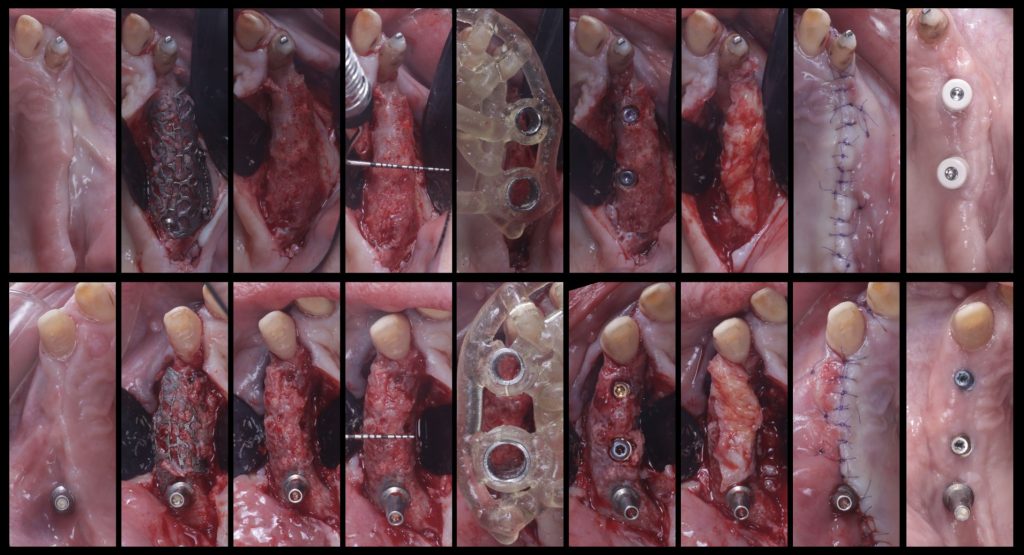
The evidence surrounding this novel treatment option is currently limited. A retrospective clinical study applying the aforementioned 3D approach reported an average vertical bone gain of 4.8 mm and a horizontal bone gain of 6.4 mm across 53 atrophic sites. The exposure rate of the meshes was reported to be 20%; however, only 5% of cases experienced partial bone loss as a result of the exposure, while the remaining 15% underwent spontaneous healing (Chiapasco et al. 2021). Furthermore, a clinical trial comparing customized vs. conventional titanium meshes in 26 patients yielded a significant reduction in average surgical time from 112 minutes to 76 minutes (p <0.01), along with a non-significant decrease in the complication rates (such as mucosal rupture and infection) to a third of their former rate (Sumida et al. 2015). Additional research has indicated that these customized titanium meshes can reliably regenerate bone volumes of around 800 mm3. It has also been shown that although complication rates were lower when the meshes were covered with a collagen membrane, the difference was not statistically significant (13% vs. 33%, p=0.39) (Cucchi et al. 2021).
Summary
The adoption of digital workflows, utilizing straightforward digital tools, is poised to enhance the predictability of our surgical procedures, thereby increasing the success rate of implantology treatment plans. Specifically, in the realm of bone augmentation, digitalization can streamline the surgical process, rendering it swifter, simpler, and more reliable. This, in turn, may result in a reduced incidence of complications, lower patient morbidity, and an improved quality of life.